How Bluetooth Works: The Hidden Tech You Use Every Day

In a world where wires are becoming a thing of the past, Bluetooth has emerged as a game-changer in the realm of wireless connectivity. This remarkable technology has not only made our lives more convenient but has also opened up a world of possibilities for device communication.
From enabling hands-free phone calls to facilitating seamless data transfer between gadgets, Bluetooth has become an indispensable part of our modern digital ecosystem.
Fundamental Technology
To grasp how Bluetooth operates, it’s essential to understand the fundamental technologies that form its foundation. At the core of Bluetooth’s functionality lies its use of radio frequency communication and its unique network architecture.
These two aspects work in harmony to enable seamless wireless connectivity between devices.
Radio Frequency Communication
Bluetooth operates in the 2.4 GHz frequency band, a portion of the electromagnetic spectrum designated for industrial, scientific, and medical (ISM) applications.
This frequency range is globally unlicensed, meaning that devices can communicate freely without the need for special permits or licenses.
To ensure reliable communication and minimize interference from other devices operating in the same frequency band, Bluetooth employs a technique called frequency hopping spread spectrum (FHSS).
FHSS involves rapidly switching the communication channel between 79 distinct frequencies within the 2.4 GHz band, with each channel occupying a bandwidth of 1 MHz.
This hopping occurs at a rate of 1,600 times per second, making it difficult for unauthorized devices to intercept or disrupt the communication.
Bluetooth divides the available channels into time slots, allowing multiple devices to communicate simultaneously without interfering with each other.
The master device in a Bluetooth network controls the timing and synchronization of these time slots, ensuring that each device transmits and receives data at the appropriate intervals.
Network Architecture
Bluetooth networks follow a master-slave architecture, where one device assumes the role of the master and controls the communication with up to seven active slave devices. This arrangement is known as a piconet.
The master device initiates the connection, determines the frequency hopping sequence, and manages the synchronization of the slave devices within the piconet.
In some cases, multiple piconets can coexist and even overlap, forming what is known as a scatternet. In a scatternet, certain devices can participate in more than one piconet by acting as a bridge between them.
These bridge devices can be either a master in one piconet and a slave in another, or a slave in multiple piconets simultaneously.
The roles of master and slave are not fixed and can change dynamically based on the needs of the devices involved.
For example, when a smartphone connects to a wireless headset, the smartphone typically assumes the role of the master, controlling the communication and managing the audio playback.
However, if the headset initiates the connection, it may temporarily become the master until the roles are renegotiated.
Connection Process
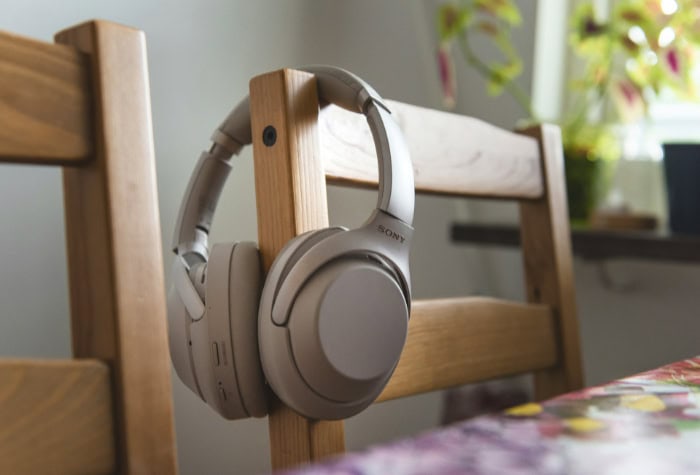
The Bluetooth connection process is a sophisticated sequence of events that allows devices to find each other, establish a secure link, and begin communication. This process involves two main stages: device discovery and pairing.
Each stage plays a crucial role in ensuring that Bluetooth devices can connect reliably and securely.
Device Discovery
Device discovery is the initial step in establishing a Bluetooth connection. During this phase, Bluetooth-enabled devices actively search for other devices in their vicinity or make themselves discoverable to others.
This process involves two distinct states: scanning and advertising.
In the scanning state, a device actively listens for signals from other Bluetooth devices that are advertising their presence. The scanning device cycles through the available frequency channels, searching for specific packets that indicate a device is available for connection.
Conversely, in the advertising state, a device broadcasts short packets of information at regular intervals. These packets contain essential details such as the device’s name, type, and sometimes the services it offers.
This information helps scanning devices identify potential connection partners.
Device identification methods vary depending on the Bluetooth version and the specific implementation. Typically, devices are identified by their Bluetooth address, a unique 48-bit identifier similar to a MAC address in other networking technologies.
In more recent Bluetooth versions, devices may also use a random address that changes periodically to enhance privacy.
Once a device has been discovered, the next step is to determine what services it offers. This is accomplished through the Service Discovery Protocol (SDP).
SDP allows devices to query each other for information about supported features and capabilities. For example, a smartphone might use SDP to determine if a nearby speaker supports audio streaming or if a printer can handle specific file formats.
Pairing Mechanism
After device discovery, the pairing process begins. Pairing is a security measure that ensures only authorized devices can connect and share data.
The pairing mechanism involves several steps to authenticate devices and establish a secure connection.
The first step in pairing is authentication. This process verifies that both devices are intentionally trying to connect with each other.
The exact authentication method can vary depending on the capabilities of the devices and the level of security required.
One common authentication method involves the exchange of a PIN (Personal Identification Number) or passkey. In older Bluetooth versions, users might need to manually enter a PIN on both devices.
Modern implementations often use a method called Secure Simple Pairing (SSP), which can generate and exchange passkeys automatically, reducing the need for user intervention.
During the PIN/passkey exchange, the devices use this shared secret to generate encryption keys. These keys are then used to secure all future communications between the paired devices.
The encryption ensures that even if someone intercepts the Bluetooth signals, they cannot decipher the transmitted data without the encryption key.
Once devices have successfully paired, they can establish a bond. Bonding refers to the process of storing the pairing information, including the shared encryption keys, for future use.
This allows the devices to reconnect quickly and securely without going through the full pairing process each time.
Bonded devices are considered trusted, and many Bluetooth implementations allow them to reconnect automatically when they come within range of each other.
This feature enhances user convenience, as paired devices like smartphones and wireless headphones can connect seamlessly without requiring user intervention each time.
Data Transmission
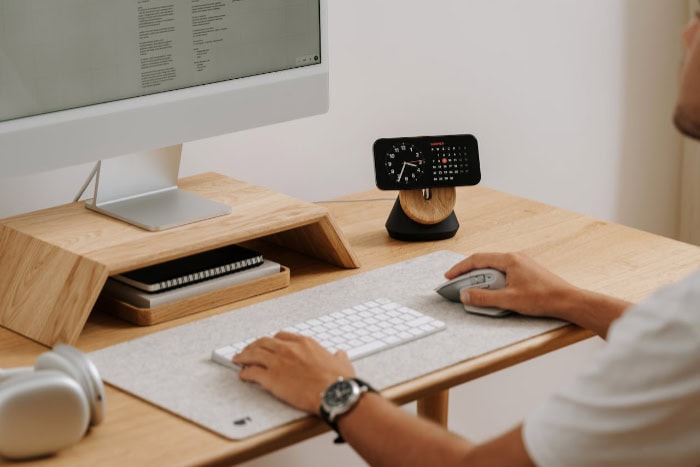
Once a Bluetooth connection is established, the real magic begins: the exchange of data between devices. To ensure that data is transmitted efficiently, reliably, and securely, Bluetooth employs a range of techniques and protocols.
From the structure of the data packets to the power management strategies, every aspect of data transmission is carefully designed to optimize performance and minimize energy consumption.
Packet Structure
At the heart of Bluetooth data transmission lies the packet structure. Bluetooth packets are the fundamental units of data exchanged between devices.
They are carefully organized and formatted to ensure that the data is transmitted correctly and can be interpreted by the receiving device.
A typical Bluetooth packet consists of several components, including an access code, a header, and a payload.
The access code is used for synchronization and identification purposes, allowing the receiving device to recognize the start of a new packet and determine which device sent it.
The header contains important information about the packet, such as its type, length, and sequence number. This information helps the receiving device process the packet correctly and maintain the proper order of data.
The header also includes fields for error detection and correction, allowing the receiving device to identify and potentially recover from transmission errors.
The payload is the actual data being transmitted, which can include anything from audio samples to sensor readings to file fragments.
The payload is often encrypted using the keys established during the pairing process, ensuring that the data remains confidential and secure.
Bluetooth uses various transmission protocols to manage the flow of packets between devices. These protocols define how devices should handle acknowledgments, retransmissions, and flow control.
For example, the Asynchronous Connection-Less (ACL) protocol is used for general data transmission, while the Synchronous Connection-Oriented (SCO) protocol is used for time-sensitive applications like voice calls.
Power Management
Efficient power management is crucial for Bluetooth devices, especially those that rely on battery power. Bluetooth technology incorporates several features and strategies to minimize energy consumption and extend battery life.
Bluetooth devices are classified into three power classes based on their maximum transmission power. Class 1 devices have the highest power output and can transmit up to 100 meters, while Class 2 and Class 3 devices have lower power outputs and shorter ranges.
By adjusting the power output based on the required range, Bluetooth devices can conserve energy when communicating over shorter distances.
In addition to power classes, Bluetooth includes various energy efficiency features. For example, devices can enter low-power sleep modes when not actively transmitting or receiving data.
These sleep modes significantly reduce energy consumption, allowing devices to conserve battery power when idle.
Bluetooth also employs adaptive power control techniques to dynamically adjust the transmission power based on the signal strength and quality.
By continuously monitoring the link conditions, devices can reduce their power output when the signal is strong and increase it when the signal is weak.
This adaptive approach helps maintain a reliable connection while minimizing energy consumption.
Furthermore, newer Bluetooth versions, such as Bluetooth Low Energy (BLE), are specifically designed for ultra-low-power applications.
BLE uses shorter data packets and operates in a sleep mode most of the time, only waking up periodically to transmit small amounts of data.
This makes BLE ideal for devices like fitness trackers, smart sensors, and other battery-powered gadgets that need to operate for extended periods on a single charge.
Security Framework
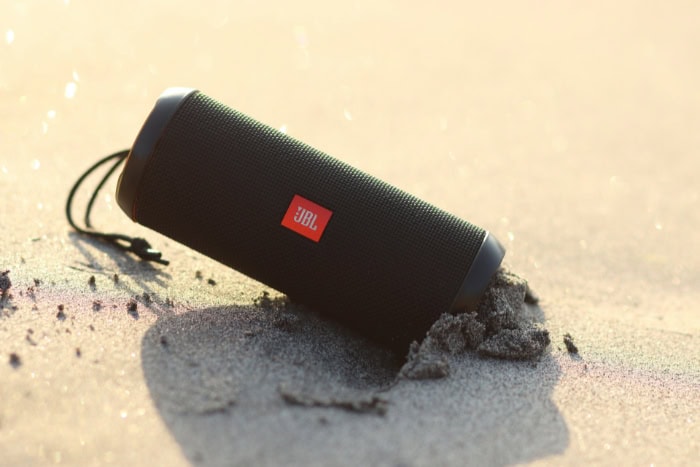
In a world where wireless communications are increasingly prevalent, ensuring the security and privacy of transmitted data is of utmost importance.
Bluetooth, being a widely adopted wireless technology, has evolved to incorporate a robust security framework that protects users’ information and prevents unauthorized access.
This framework consists of advanced encryption methods and privacy protection measures that work together to create a secure environment for Bluetooth connections.
Encryption Methods
Encryption is the foundation of Bluetooth security, ensuring that data transmitted between devices remains confidential and protected from eavesdropping.
Over the years, Bluetooth has adopted increasingly sophisticated encryption methods to keep pace with evolving security threats.
One of the most significant advancements in Bluetooth security is the introduction of Secure Simple Pairing (SSP). SSP is a key agreement protocol that simplifies the pairing process while enhancing security.
It uses a combination of public-key cryptography and passkey authentication to establish a secure connection between devices. SSP offers four different association models, each tailored to specific device capabilities and user interaction requirements.
Once a secure connection is established, Bluetooth employs the Advanced Encryption Standard (AES) with Counter with CBC-MAC (CCM) mode for data encryption.
AES-CCM provides strong encryption and message authentication, ensuring that data remains confidential and unaltered during transmission.
The encryption keys used in this process are derived from the link key generated during the pairing process, making each connection unique and secure.
The key generation and exchange process is designed to prevent eavesdropping and man-in-the-middle attacks.
Bluetooth uses the Elliptic Curve Diffie-Hellman (ECDH) key agreement protocol, which allows devices to securely generate and exchange encryption keys over an untrusted channel.
This ensures that only the intended devices can access the shared secret key and decrypt the transmitted data.
Privacy Protection
In addition to encryption, Bluetooth incorporates several privacy protection measures to safeguard users’ personal information and prevent unauthorized tracking. These measures help mitigate the risks associated with wireless communications and enhance user privacy.
One important privacy feature is address randomization. Bluetooth devices are identified by their unique MAC addresses, which can potentially be used to track users’ locations and movements.
To mitigate this risk, Bluetooth introduced the concept of random resolvable private addresses (RPA). These dynamically generated addresses change periodically, making it difficult for attackers to track devices over time.
Only devices with the appropriate encryption keys can resolve these private addresses and establish connections.
Bluetooth also defines different levels of connection security, allowing developers to choose the appropriate level based on the sensitivity of the transmitted data.
The four main security levels are “no security,” “unauthenticated pairing with encryption,” “authenticated pairing with encryption,” and “Authenticated LE Secure Connections pairing with encryption.”
By selecting the appropriate security level, developers can balance the need for protection with the requirements of their specific application.
To further enhance privacy and security, Bluetooth continuously evolves to address newly discovered vulnerabilities and threats. The Bluetooth Special Interest Group (SIG) regularly releases updates and security patches to mitigate known issues and strengthen the overall security framework.
Performance Characteristics
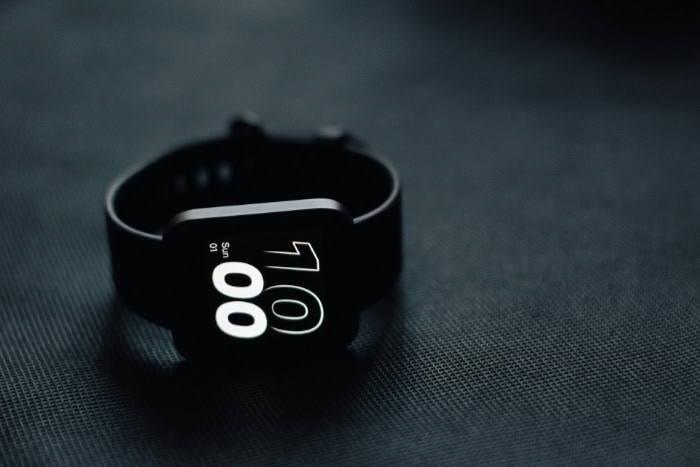
The success of Bluetooth as a widely adopted wireless technology can be attributed, in part, to its impressive performance characteristics. From its speed and range to its ability to manage interference, Bluetooth is designed to deliver reliable and efficient wireless communication in a variety of environments.
Speed and Range
One of the most critical aspects of Bluetooth performance is its data transfer rate. The speed at which data can be transmitted between devices determines the responsiveness and quality of the user experience.
Over the years, Bluetooth has evolved to offer increasingly higher data rates, enabling more demanding applications such as high-quality audio streaming and large file transfers.
This increased bandwidth allows for faster data transmission and reduces latency, resulting in a more seamless and responsive user experience. However, it’s important to note that actual data throughput may be lower due to protocol overhead and other factors.
In terms of operating distance, Bluetooth is primarily designed for short-range communication. The typical range for Bluetooth devices is around 10 meters (33 feet), although this can vary depending on the device’s power class and antenna design.
Bluetooth 5.0 introduced an optional long-range mode that can extend the range up to 240 meters (800 feet) in open areas, but this comes at the cost of reduced data rate and increased power consumption.
Environmental factors can also significantly impact Bluetooth’s speed and range. Obstacles such as walls, furniture, and human bodies can attenuate the signal and reduce the effective range.
Similarly, interference from other electronic devices and wireless networks can degrade performance and cause data loss. To mitigate these issues, Bluetooth devices often incorporate power control and adaptive frequency hopping mechanisms.
Interference Management
In today’s crowded wireless landscape, interference management is crucial for ensuring reliable and consistent Bluetooth performance.
With numerous devices and technologies competing for the same 2.4 GHz frequency band, Bluetooth must employ sophisticated techniques to coexist with other wireless networks and minimize interference.
One of the primary methods Bluetooth uses to manage interference is adaptive frequency hopping (AFH). AFH allows Bluetooth devices to dynamically adjust their frequency hopping pattern based on the presence of other wireless signals in the environment.
By avoiding channels that are occupied by other devices, such as Wi-Fi networks or cordless phones, Bluetooth can minimize interference and maintain a stable connection.
Bluetooth also incorporates various channel hopping algorithms to further enhance its resilience to interference. These algorithms determine the order and timing of frequency hops, ensuring that devices can quickly adapt to changing channel conditions and maintain a reliable link.
In addition to channel hopping, Bluetooth employs signal optimization techniques to improve performance in noisy environments.
These techniques include adaptive power control, which adjusts the transmission power based on the signal strength and quality, and forward error correction (FEC), which adds redundancy to the transmitted data to enable error detection and correction.
Bluetooth’s interference management capabilities have been further enhanced in recent versions through the introduction of new features such as slot availability mask (SAM) and periodic advertising.
SAM allows Bluetooth devices to communicate their available time slots to other devices, enabling more efficient channel utilization and reducing the likelihood of collisions.
Periodic advertising, on the other hand, enables devices to transmit data at fixed intervals, minimizing the impact of interference from other devices.
Conclusion
Bluetooth’s intricate workings reveal a technology that has masterfully balanced complexity and user-friendliness. From its radio frequency foundations to its sophisticated security measures, Bluetooth exemplifies the art of wireless communication.
The technology’s ability to adapt and thrive in an increasingly crowded wireless spectrum is a testament to its robust design and ongoing development.
Looking beyond the technical aspects, Bluetooth’s impact on our daily lives is profound. It has silently woven itself into the fabric of our digital interactions, enabling everything from hands-free calling to smart home automation.